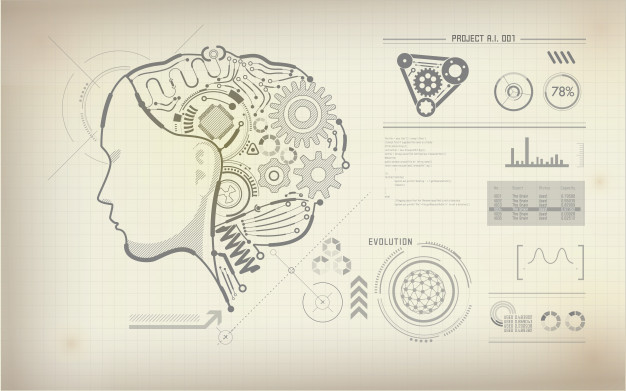
The human body is indisputably the most obscure ‘natural apparatus’ on the planet! As healthcare systems around the globe grunt under the weight of the strain, the data-intensive nature of healthcare makes it an ideal candidate for the application of AI across multiple disciplines, from diagnosis and pathology to drug discovery and epidemiology. Looking at the fact that about ninety percent of all healthcare data is derived from imaging technology (of which the bulk has not been analyzed) it seems that AI’s impact is most prevalent in healthcare as the technology matures. Alzheimer’s, eye disease, cancer, X-rays – no area of medicine is left untouched by the power of machine intelligence. Healthcare’s reliance on large amounts of data makes medical diagnostics one of the most exciting yet most dangerous fields of AI.
Biomedical engineering (BME) is specialized bioengineering (the study of applied engineering practices in general biology) plus putting into practice many of the field’s basic theories and techniques to advance human health and health care. Skills needed to be a biomedical engineer are attention to detail, measurement and analytical skills, an eye for design, technical and creative skills (the ability to create products from designs), the skills to communicate and work in a team, and being able to empathize with patients. In an undergraduate program, a BME will be exposed to differential equations, calculus, calculus-based physics, linear algebra, and statistics. Due to an increase in the possibilities of new technologies and increased applications of medical devices and equipment, from 2016 to 2026, the employment of BMEs is set to grow as rapidly as the average of all other occupations. When NASA searched for a novel group of astronauts, only 12 were selected from more than 18,000 applicants.
NASA jobs are difficult to come by and tough to keep, but NASA is the place to stop if you are looking for an exceptional job. To work for a NASA service provider you do not have to be more skilled than for any other engineering firm.; although, you should have a bachelor’s degree in engineering, computer science, mathematics, biological science, or physical science (such as chemistry, geology, or physics) for example a Bachelor of Engineering Science in Biomedical Engineering, BEngSc (BME), which combines specific biomedical engineering courses with subjects in engineering, science, biology, and medicine. Further, you should have three years of professional experience (or 1,000 hours of pilot-in-command time in a jet aircraft) and pass NASA’s physical examination for astronauts. To work at the bioengineering branch of NASA, you have to invent advanced life-support machines for astronauts to breathe longer in space. Currently, they have limited time before they need to return to the craft (they have to refill their life-sustaining machines). I must add that BEngSc (BME) is a pre-professional qualification – you are not entitled to professional registration based on the degree alone. After your bachelor’s degree, you have various options to acquire a professional qualification such as BSc(Eng) in Electrical or Information Engineering, Medicine (MBBCh), and BSc(Hons) in Physics.
As BME provides an extensive understanding of disease processes, it applies engineering and other quantitative sciences to solve biological and medical problems such as to develop medical devices, image recognition systems, refined X-ray imaging systems, and artificial organs. Biomedical engineers have knowledge of engineering, mechanics, biology, physiology, health informatics, and healthcare. They can combine their different skills to construct results that address global health issues, to lower the cost of healthcare, and to alter the way patients are treated.
First of all (together with chemists and medical- and life scientists), a biomedical engineer carries out research regarding the engineering aspects of the biological systems of animals and humans. In a hospital setting, a BME provides advice on the choice and use of medical equipment and builds tailored devices for research and special health care needs. The job includes testing medical products for effectiveness and safety, testing medical equipment, designing artificial organs, developing blood sensors, creating advanced imaging devices, as well as therapeutic methods for injury recovery. Firstly, a BME develops electrical circuits, tests new drug therapies via computer models, or designs software to run medical equipment. Secondly, a BME builds artificial body parts such as knee and hip joints as well as substances to create replacement body parts. During all this, a BME faces the challenge to uphold patient privacy!
The Biomedical Engineering Society (BMES) is the professional home of more than 7,500 global bio- and biomedical engineering members who form the collaborative bond between engineering and medicine. The purpose of Special Interest Groups in the research areas of BMES, other than to provide BMES with direct access to members, is to allow members to develop within a professional setting, to supply members with a forum to exchange ideas and information, and to provide network opportunities to discuss issues. The BMES Medical Devices Special Interest Group (SIG) was established as part of a new partnership between BMES, industry, and the US Food and Drug Administration (FDA). The SIG will promote collaboration between industry, FDA, regulatory professionals, academic researchers, clinicians, practicing engineers, and students interested in discovering the art of designing, testing, and the use of medical devices to improve the lives of patients around the globe. In a SIG Meeting of BMES, the FDA held a public workshop (April 2020) at their White Oak Campus in Silver Spring, Maryland, US named Towards Good Simulation Practices in Health Technologies. The purpose of the workshop was to develop a framework to evaluate and assess the role of computational modeling in health technologies and to discuss modeling practices currently used by industry in health technologies.
BMES produces several scientific journals to keep its members informed of activities within BMES as well as developments within the biomedical engineering professions. These journals are the monthly Annals of Biomedical Engineering (original research in areas such as biomechanics and molecular motion, biological signal processing and instrumentation, biomaterials and biological interfaces, regulatory and integrative biology, cellular engineering and biotechnology, systems analysis and electrophysiology), Cardiovascular Engineering and Technology (CVET) which offers an academic and industrial forum to share research about the cardiovascular system and present research in all facets of cardiovascular physiology and medical treatment regarding engineering theories and techniques to advance essential knowledge and technological explanations, Cellular and Molecular Bioengineering (the main challenge in improving human health is to understand how cellular behavior starts from molecular-level interactions. It searches for answers to the relation between the cellular and molecular levels to eventually manage the chemical, electrical, and mechanical processes of the cell – experimental and theoretical studies to advance knowledge such as the biomechanics of cells and their interaction with the extracellular environment), as well as Biomedical Engineering Education which presents academic articles and announcements regarding education, practice and scholarships in bioengineering, biomedical engineering, and their allied fields; it presents a range of topics such as the professional development of BME students, engineering education research, and laboratory demonstrations).
BMES has answered some nagging questions about BME. Biomedical engineers bridge the medical and engineering disciplines to provide a refurbished health care system. BME tasks are such as sports medicine – rehabilitation, or external support devices; blood chemistry sensors to measure potassium, sodium, O2, CO2, or pH); the use of expert systems and AI for clinical decisions – computer-based systems to diagnose diseases; automated patient monitoring during surgery or in intensive care, healthy persons in unusual environments, astronauts in space or underwater divers at great depth; advanced therapeutic and surgical devices – a laser system for eye surgery, or automated delivery of insulin; artificial organs – artificial hearts and kidneys, blood oxygenators, cardiac pacemakers, hearing aids, or synthetic blood vessels, arms, joints, and legs; biomechanics of injury and wound healing – application of growth factors, or gait analysis; biomaterials design – biocompatibility properties of implantable artificial materials; computer modelling of physiologic systems – (blood pressure control, renal function, or visual and auditory nervous circuits; design of optimal clinical laboratories – cardiac catheterisation laboratory, or computerised analyser for blood samples; as well as medical imaging systems – computer assisted tomography, magnetic resonance imaging, positron emission tomography, or ultrasound.
As part of the government’s Industrial Strategy Challenge to improve healthcare, five new AI research centres were declared last year in the UK in Coventry (central England), Glasgow (Scotland), Leeds (Yorkshire), Oxford (Oxfordshire), and London. The main focus of these centres will be on the rapidly advancing area of image analysis namely the two disciplines of radiology (the science of medical imaging) and histopathology (changes in tissues caused by disease). Medical diagnostics can be reshaped by applying AI across these two fields for example diagnosis from a single X-ray varies from infectious diseases to cancers.
The ultimate goal is to train AIs across multiple diseases to suggest a potential diagnoses from an X-ray. The prospect is to have a computer algorithm to assist the human professional in situations of difficult diagnoses; AIs will have an assistive role and will not replace the medical professional. Already at work is Google’s AI company DeepMind (founded during 2010); since 2016, DeepMind Health and London’s Moorfields Eye Hospital have been using routinely collected images to prematurely diagnose degeneration such as training software to diagnose ocular conditions from digitised retinal scans. The result was an AI system that is able to recommend the correct referral decision for over 50 eye diseases with more than ninety percent accuracy (which equals the performance of peak medical experts). Although its technology delivered remarkable results, its data policy and intimate union with Google is a cause of concern; companies have a duty of transparency in their use of data as well as to protect it. Sick people may be happy for their data to be used, but, there is a responsibility to keep their data confidential.
Early diagnosis of cancer can radically improve survival rates. GE Healthcare is one of a group of outstanding industry partners that want to connect data as an element of the UK’s new imaging project; it forms part of the National Consortium of Intelligent Medical Imaging (NCIMI) which aims to use AI for earlier diagnosis, intentional treatment, and personalised care. In the fast-paced world of start-ups, various radiology techniques are exposed to AI. Lancor Scientific is at the rear of a new diagnostic device that (combined with AI) can alter the method of cancer detection. The underlying technology, namely Opto-magnetic Imaging Spectroscopy (OMIS), is supported by the mathematical equations of Maxwell which is based on electromagnetism; when light shines onto human tissue, the magnetic element of the reflected light will determine how malignant the cancer is. With a disease such as Alzheimer’s, early detection has more to do with management than survival. Given the fact that Alzheimer’s does not have a cure, the ageing population with dementia will triple by the year 2050. In the interest of global health, the phenomenon of dementia has been expressed as ‘a ticking time bomb.’