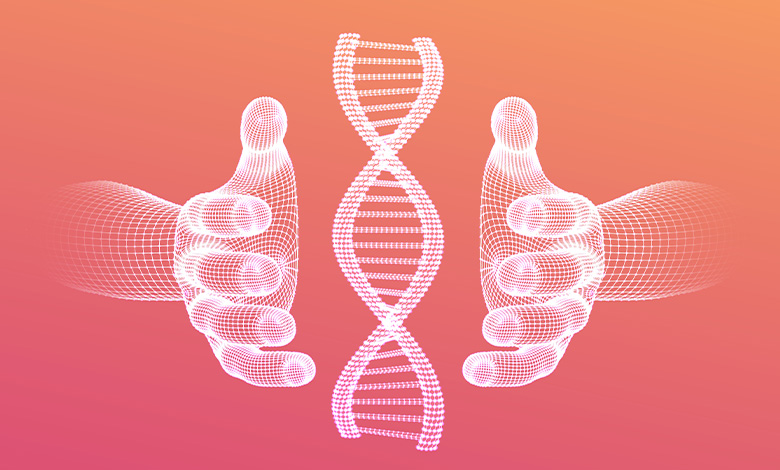
Engineering has amalgamated with Chemical Engineering (CE) to include various sub-categories such as Biochemistry (BC), Biotechnology (BT), Bio or Biomedical Engineering (BE), Biochemical engineering (BCE), Bioelectrical engineering (BEE), and Biomolecular Engineering (BME). These are my own abbreviations to optimistically uncomplicate the many sub-divisions of each sub-category. It is the latter sub-division, which deals with the manipulation of primary biomolecules that I want to concentrate on.
These biomolecules include carbohydrates, lipids, nucleic acids, and proteins. They are the basic building blocks of life and to create, control, and manipulate them brings benefits, improvements, and fresh fields of learning to humanity. For students of undergraduate studies it promises new approaches to a chemical engineering program. According to the Accreditation Board for Engineering and Technology (ABET), biomolecular engineering ‘must provide thorough grounding in the basic sciences including biology, chemistry, and physics.’ A Bachelor of Science degree in engineering includes courses such as separation, transport, thermodynamics and kinetics, as well as life sciences such as biology and biochemistry (which includes specialised biomolecular courses that focus on biopolymers, cell biology, nano- and biotechnology).
CE is applied chemistry. It is the branch of engineering that deals with chemical production and the manufacture of products through chemical processes; the processing of raw materials to produce reactants, the separation of products, the recycling of by-products, and the disposal of wastes. Like science, it starts in the laboratory, but (through the design and implementation phase) it develops into a full-scale process. Each stage involves basic building blocks (or unit operations) such as extraction, filtration, and distillation. A Dictionary of Chemical Engineering by Carl Schaschke is the most authoritative of its kind. It not only provides definitions and explanations for chemical engineering terms, but includes terms from the fields of biology, chemistry, physics, and mathematics.
BT includes applications such as biochip, bioelectronics, and biosensor (engineered devices and systems to measure, monitor and control biological processes), biomaterials (design, synthesis and production of new materials to sustain cells and tissues), bioprocess engineering (design and maintenance of cell- and enzyme-based processes to manufacture fine chemicals and pharmaceuticals), and genetic engineering (decisive manipulation of the genomes of organisms to produce new phenotype features).
BE is a wide term which includes all engineering applied to the life sciences. It uses the principles of biology in conjunction with the principles of engineering to create viable products. Applications of bioengineering are processes such as biomimetics (the study and development of synthetic systems to imitate natural biologically produced processes and substances), bioprocess engineering (the study and development of process equipment and optimisation to aid in the production of products such as foods and pharmaceuticals), and industrial microbiology (the execution of microorganisms to produce industrial products such as foods and antibiotics, as well as the use of microorganisms to treat wastewater in chemical plants).
BCE includes applications such as biocatalysis (chemical transformation with enzymes), bioreactor design and analysis (the design of reactors to execute biochemical transformation), bioseparations (the separation of biologically active molecules), and thermodynamics and kinetics – chemistry (the analysis of reactions that engage cell growth and biochemicals).
BEE involves the electrical fields produced by living cells or organisms. An example is the electric ability developed between nerves or muscles of the body. Knowledge of biology and electricity is required to improve current bioprocesses or technology. Applications are such as bioelectrochemistry (chemistry of electron/proton transport through the cell) and bioelectronics (research that links biology and electronics).
Biomolecular Engineering (BME)
The need for penicillin during World War II brought together chemical engineers and microbiologists. Much later in time it led to a chain reaction of research in the fields of biology and chemical engineering (to study the molecular structure). The field was defined by the US National Institutes of Health during 1992, which changed the ‘research’ label to ‘biomolecular engineering’; the journal New Biotechnology had the previous name of Biomolecular Engineering. Because BME is now a widespread discipline with applications in many fields and industries, it is difficult to gain perspective of the profession; however, the field of biotechnology (biotech) includes all firms that use biotechnology to perform biotechnological research and development or to produce goods or services. Thus, it includes industrial applications in the biomolecular engineering field.
Allow me to further explain BME. It uses a lot of similar principles and practices as bioengineering, but focuses on medical applications and decidedly manipulates molecules of biological origin. Applications of BME are such as artificial organs (the application of tissue engineering to whole organs), biomaterials (the design of new materials for implantation in the human body and analysis of their effect on the body), cellular engineering (the design of new cells using recombinant DNA and the development of procedures to allow normal cells to adhere to artificial implanted biomaterials), human factors and ergonomics (the design of systems to improve human performance), man-machine interfacing (the control of surgical robots and remote therapeutic and diagnostic systems using voice recognition, eye tracking, and muscle and brain wave controls), medical imaging (the imaging of tissues using CAT scan, MRI, ultrasound, x-ray or other technologies), medical optics and lasers (the use of lasers for medical diagnosis and treatment), rehabilitation engineering (the design of gadgets and systems to assist the disabled), and tissue engineering (the design of new tissues from basic biological building blocks to create new tissues).
Due to its maturity in the analyses of gene patterns and the decisive manipulation of significant biomolecules to improve functionality, BME has the ability to become a vital scientific discipline. Research in BME can lead to improved therapies, new drug discoveries, and the development of new bioprocess technology. As the knowledge of biomolecules augments, the speed of discovery of quality novel molecules (which will include antibodies, enzymes, vaccines, and therapeutic peptides) increases. Using recombinant technology, new antibiotics that are active against resistant strains, can be produced.
AI research and the COVID pandemic
Medical images provide vital information to detect, diagnose and monitor diseases such as COVID-19. X-rays or CT scans of the lungs can assist doctors to determine the severity of the disease and to decide on the best treatment; automated systems have the ability to improve the accuracy and time output. For artificial intelligence to truthfully examine a scan, thousands of images must be collected to instruct machine-learning algorithms.
The NIH (the US medical research agency with 27 institutes and centers) is part of the US Department of Health and Human Services. NIH is the prime federal agency responsible for the performance and maintenance of basic and clinical medical research, and to investigate the causes, treatments, and cures for common and rare diseases. The NIH has launched an ambitious effort to exploit the power of artificial intelligence and medical imagery in the fight of COVID-19. The multi-organisational collaboration (led by NIBIB) will create new tools to detect and treat COVID early.
The National Institute of Biomedical Imaging and Bioengineering’s (NIBIB) mission is to develop, improve, and speed health through biomedical technologies. The Institute will amalgamate engineering and physical science with biology and medicine to detect, diagnose, treat, and prevent disease. According to Bruce J. Tromberg, Ph.D. (NIBIB Director) ‘this program unites leaders in medical imaging and artificial intelligence from academia, professional societies, industry, and government to take on this important challenge.’ Consistent with Krishna Kandarpa, M.D., Ph.D. (director of research sciences and strategic directions at NIBIB) ‘this major initiative responds to the international imaging community’s expressed unmet need for a secure technological network to enable the development and ethical application of artificial intelligence to make the best medical decisions for COVID-19 patients. Eventually, the approaches developed could benefit other conditions as well.’ Guoying Liu, Ph.D., (the NIBIB scientific program lead on the COVID effort) says ‘it will gather a large repository of COVID-19 chest images, allowing researchers to evaluate both lung and cardiac tissue data, ask critical research questions, and develop predictive COVID-19 imaging signatures that can be delivered to healthcare providers.’
A new center, The Medical Imaging and Data Resource Center (MIDRC), launched and funded under the NIH’s emergency COVID-19 program), and hosted at the University of Chicago (Pritzker School of Medicine), will have an enormous database of medical images to enable improved understanding and treatment of COVID. According to Kenneth S. Polonsky (Dean of the Division of the Biological Sciences and Pritzker School of Medicine) the University of Chicago is fit to host the MIDRC ‘as members of our faculty have led the development of data commons repositories in the past’ which includes the National Cancer Institute’s Genomic Data Commons. Prof. Maryellen L. Giger PhD, (the A.N. Pritzker Professor of Radiology, Committee on Medical Physics, UC), will co-lead with an executive advisory committee that includes members of the American College of Radiology (ACR) namely Etta Pisano, MD, and Michael Tilkin, MS, the Radiological Society of North America (RSNA) namely Curtis Langlotz, MD, PhD, and Adam Flanders, MD, and the American Association of Physicists in Medicine (AAPM) namely Paul Kinahan, PhD. Collaboration among the ACR, RSNA, and AAPM is based on each organisation’s expertise and dedication within the medical imaging community.
MIDRC offers a platform to collect, store and share COVID-related medical imagery to enable effective analyses and clinical distribution. This will involve 5 development projects (under the supervision of the MIDRC executive advisory committee) which include launching a portal for data access and distribution, and linking the MIDRC to other clinical and research data registries. The committee will direct and fund 12 research projects, support investigators of the ACR, RSNA and AAPM, and sustain universities. The MIDRC aims to upload more than 10,000 COVID-19 thoracic radiographs and CT scan images via the existing portals of the RSNA and the ACR registry (within 90 days), allowing researchers across the globe to access the images and to have clinical data answers to new questions about the disease. The key test is to rapidly and accurately identify algorithms and calculate the information when combined with numerous other clinical symptoms and tests. While COVID will be the primary focus, it will not be the only objective.
According to Dr. Giger, the MIDRC’s Data Commons Portal will give investigators access to images and data, speed up research and provide solutions to the COVID-19 pandemic. ‘We have not sufficiently explored imaging for its role in helping us fight COVID-19, especially in terms of developing machine intelligence tools and systems. But having these top imaging organisations involved will make a difference—almost every scientist or clinician in medical imaging belongs to at least one of these organisations. Ultimately, it will be expanded to incorporate additional data from multiple registries and repositories to support the NIH’s data collection efforts, allowing researchers to address topics no single archive could inform independently.’